How RocSlope2 Validated Real-World Wedge Failure Analysis in Montenegro
In Tivat, Montenegro, engineers investigated the stability of a 15 m-high excavation slope in layered limestone, where intersecting joint sets posed a clear wedge failure risk. They aimed to understand how seismic loading, water pressure, and different anchoring strategies affect the safety factor of the slope.
Originally conducted using SWedge, we recreated the research using RocSlope2 to validate its ability to replicate and extend the same deterministic wedge analysis. Below, we’ll cover the initial research findings and show how RocSlope2 matched the original results across all scenarios. You can also read the full research here.
The Geological Setting
In Tivat’s mountainous coastal region, engineers assessed the risk of wedge failure in stratified limestone slopes to better understand the area’s rockfalls and instability, as they can impact building, bridge, dam, and mine construction. The site in question — a 15 m-high excavation slope with steep geometry and intersecting joint sets — presented textbook conditions for instability. With excavation slopes trending at 250° and dipping 82°, and joint families identified at 145°/80° and 220°/65°, the possibility of wedge formation was evident.
In an environment prone to both heavy rainfall and seismic activity, the engineering team needed to quantify how wedge stability would be affected not just by geometry, but also by water pressure, seismic loading, and the type of reinforcement used.
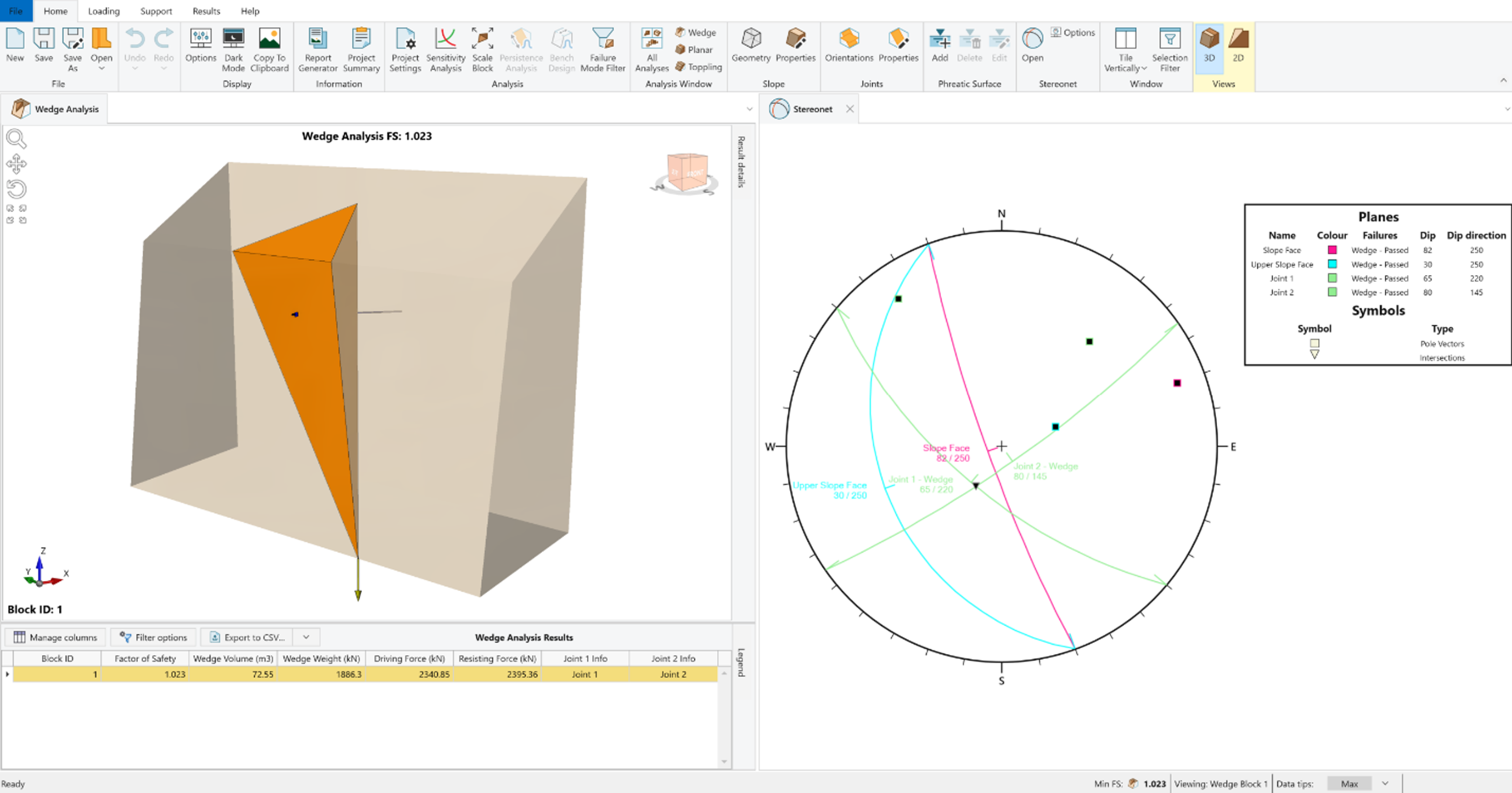
The Challenge
Researchers set out to analyze how various real-world factors, such as partial water pressure in the joints and seismic loading, influence wedge stability in a steep limestone slope, moving beyond basic dry and static conditions to model more complex scenarios. They needed to understand how different reinforcement strategies, specifically active versus passive anchors, would affect the safety factor.
In addition, they examined how the choice of joint shear strength model, comparing the linear Mohr-Coulomb criterion with the nonlinear Barton-Bandis model, could significantly alter design outcomes and required stabilization forces.
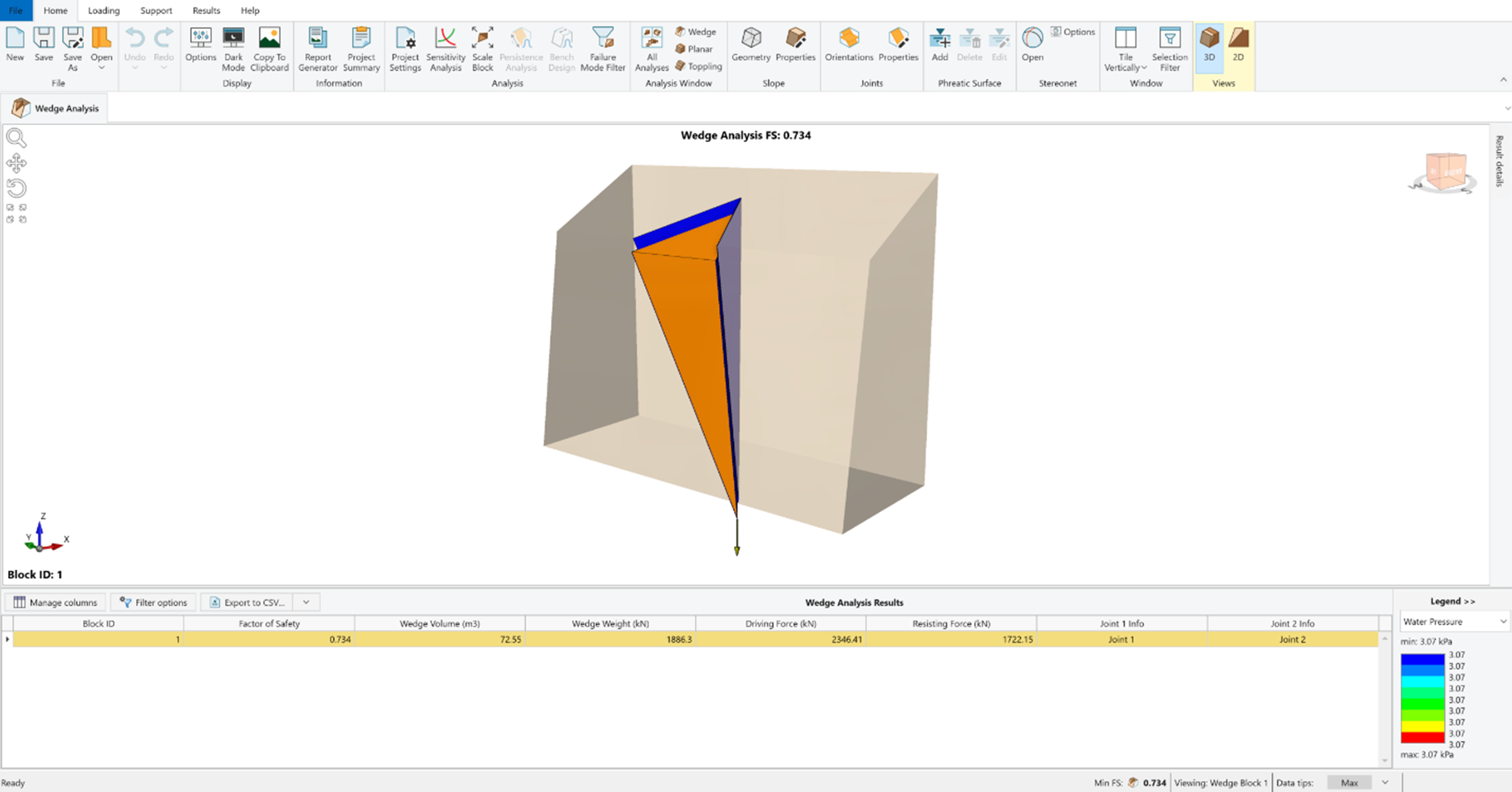
The Solution
The original analysis was carried out in SWedge, where the team modelled wedge geometry, water pressure, seismic loading, and anchor performance using field data and stereographic projections from Dips. These scenarios revealed how quickly safety factors drop under seismic conditions — and how much force is needed to stabilize the slope.
The model was reconstructed in RocSlope2 to take advantage of its modern analysis tools and enhanced interface. The results matched exactly, demonstrating RocSlope2’s effectiveness in analyzing these conditions.
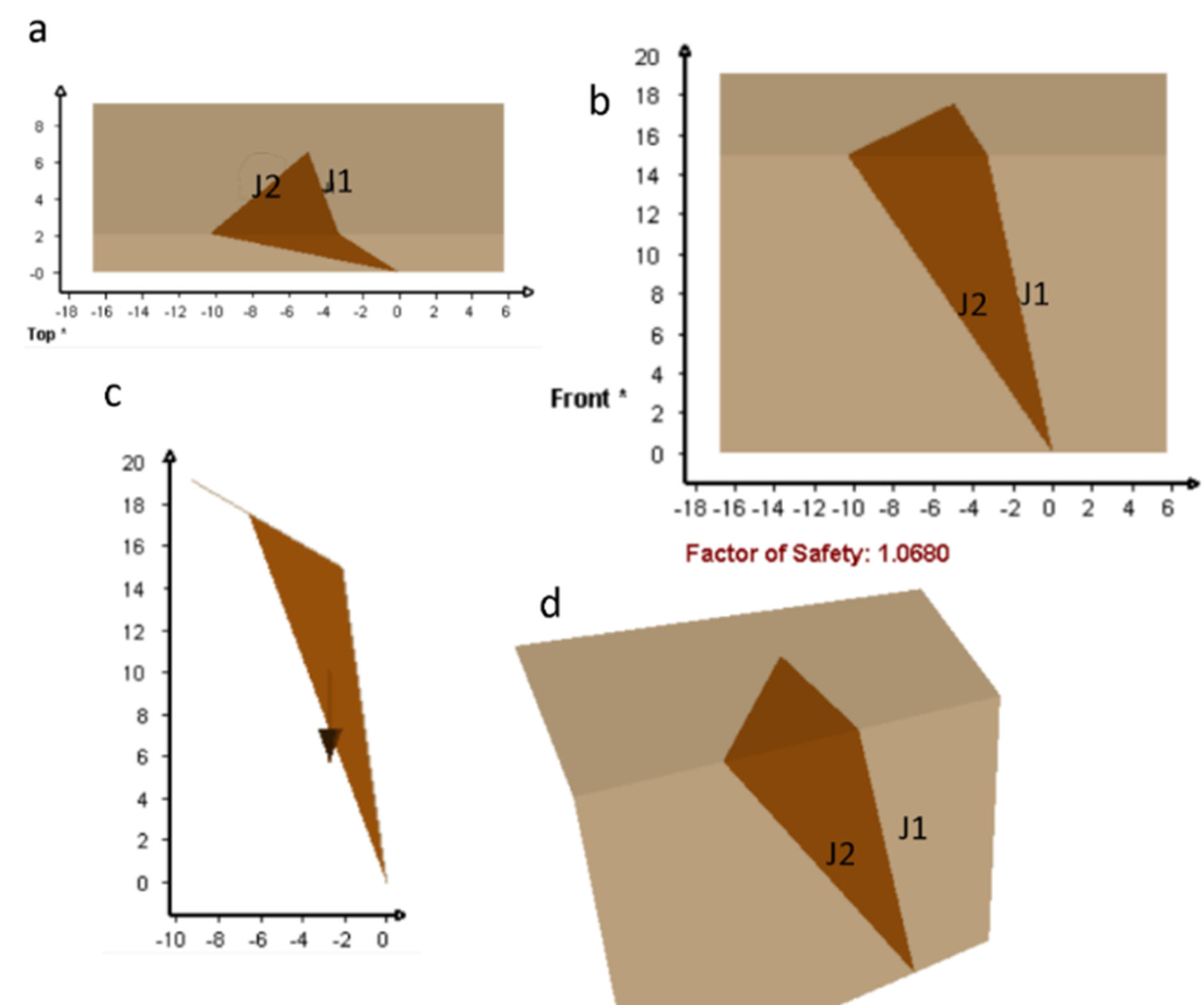
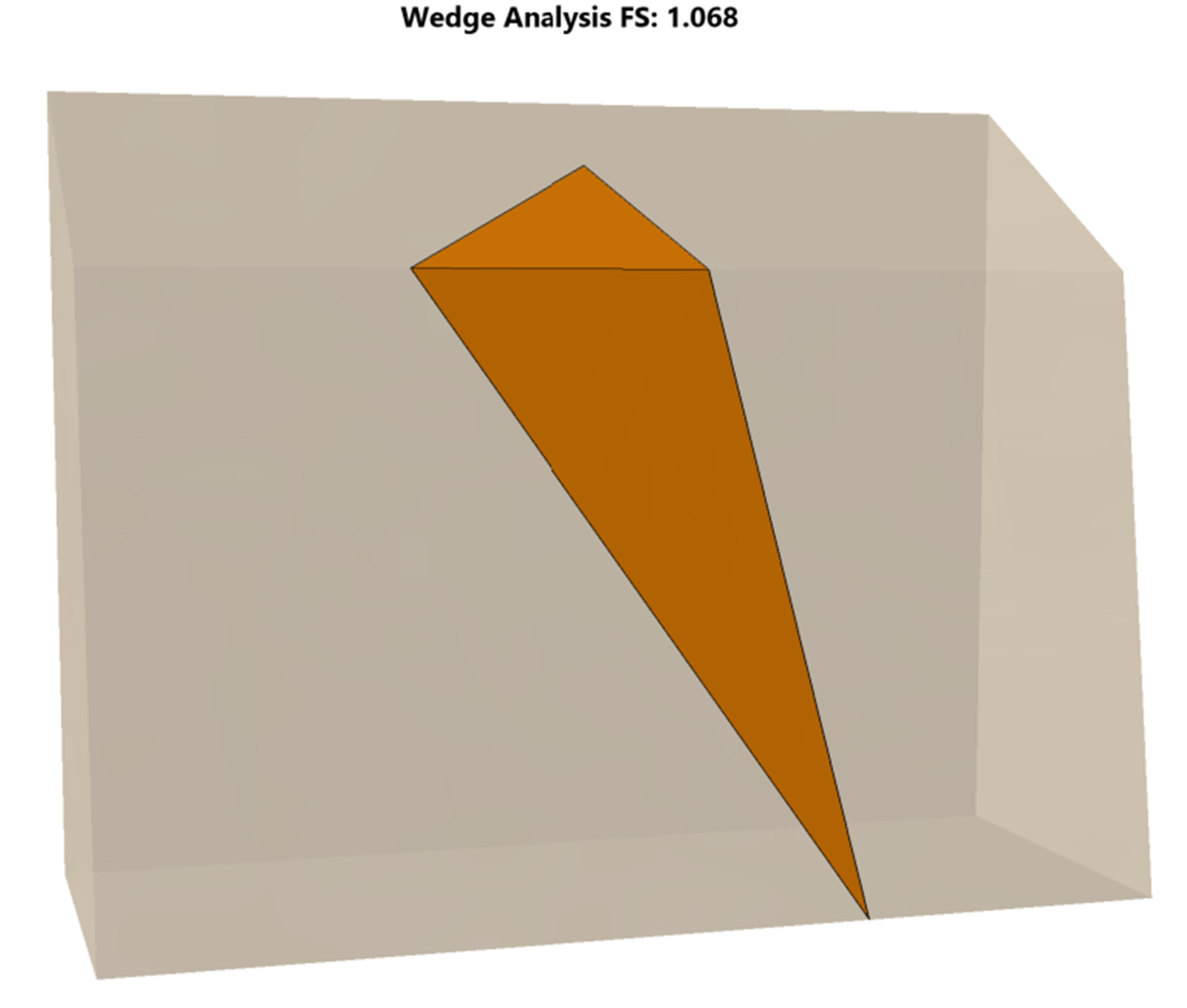
The Results
The parametric study revealed just how sensitive wedge stability is to additional water pressures in the joints, seismic forces and reinforcement design. Without anchors, the wedge was unstable under both static and seismic conditions (Fs = 0.99 in wet conditions, Fs = 0.78 under seismic loading). Active anchors drastically improved performance. A total anchor force of 200 kN raised the safety factor to Fs = 1.55 in dry-static and Fs = 1.12 under seismic loading.
In contrast, passive anchors needed a total force of 800 kN to reach Fs = 1.02 under the same seismic conditions — four times the load required by active systems. When the Barton-Bandis failure criterion was applied instead of Mohr-Coulomb, required anchor forces quadrupled to reach Fs = 1.00 under seismic loading, showing the design implications of using a nonlinear Barton-Bandis shear strength model.
RocSlope2’s ability to run these comparisons quickly, with intuitive 3D visualizations and customizable anchor parameters, makes it possible to explore a wide range of conditions without sacrificing rigour.
Case |
Strength Type |
Water Pressure Applied |
Seismic Coefficient kh |
Anchor Capacity (kN) |
SWedge |
RocSlope2 |
1 |
Mohr-Coulomb |
No |
0 |
- |
1.07 |
1.07 |
2 |
Yes |
0 |
- |
0.99 |
1.00 |
|
3 |
No |
0.335 |
- |
0.78 |
0.78 |
|
4 |
Yes |
0.335 |
- |
0.73 |
0.73 |
|
5 |
No |
0 |
200 (Passive) |
1.55 |
1.55 |
|
6 |
No |
0.335 |
200 (Passive) |
1.12 |
1.12 |
|
7 |
Yes |
0.335 |
550 (Passive) |
1.20 |
1.22 |
|
8 |
Barton-Bandis |
No |
0.335 |
800 (Passive) |
1.00 |
1.00 |
9 |
Mohr-Coulomb |
No |
0.335 |
800 (Active) |
1.02 |
1.02 |
Table 1. An overview of the wedge stability analysis conducted. Note the factors of safety on the right, where the original data from SWedge is recorded alongside the validated results in RocSlope2.
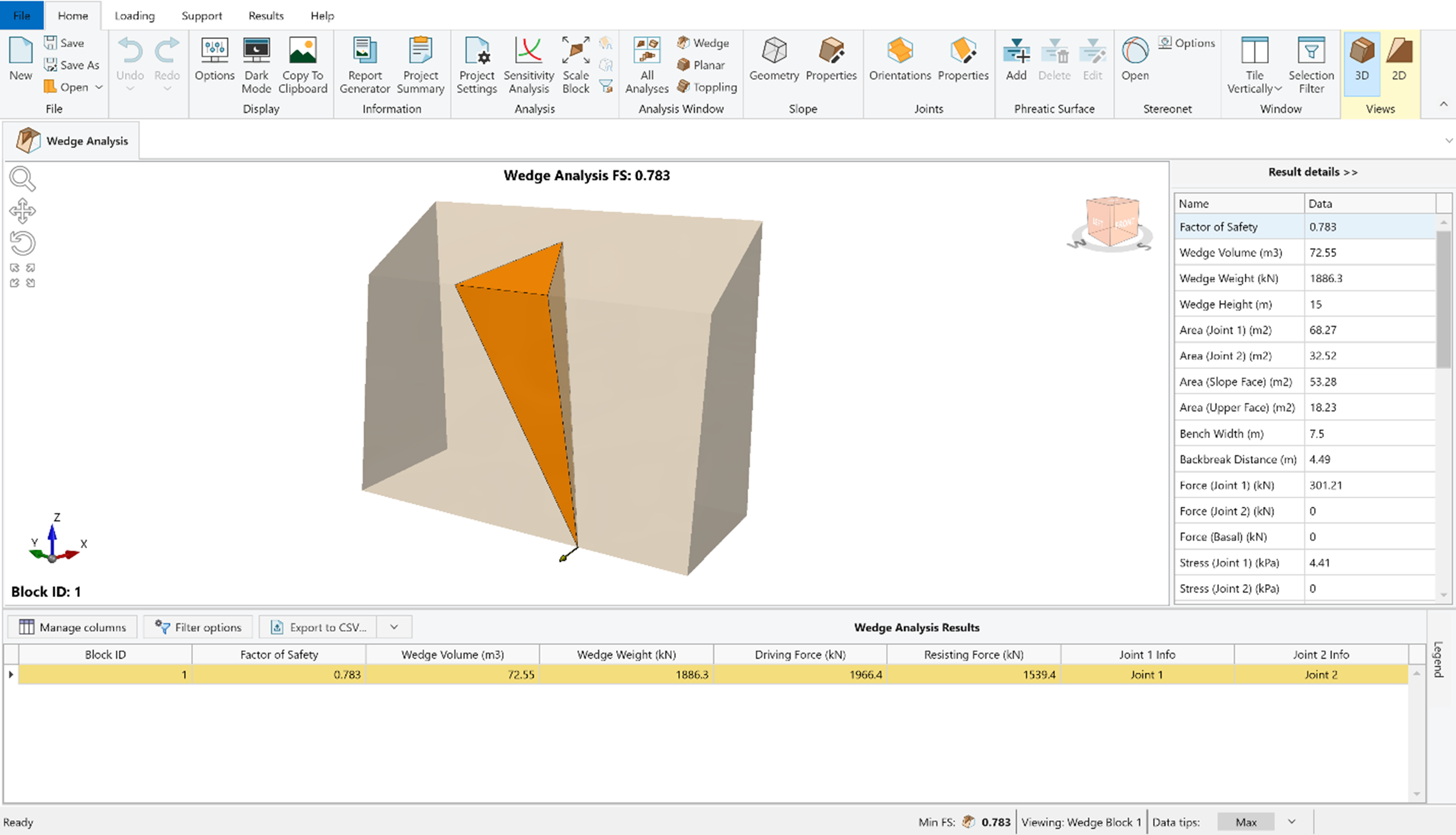
The Verdict
Modern rock slope design requires more than basic geometry checks. When seismic forces, water pressure, and various joint shear behaviors are at play, RocSlope2 provides essential insight into how wedges form — and how to stabilize them efficiently.
By replicating the original model from SWedge, RocSlope2 proved to be just as reliable and powerful for deterministic wedge analysis. Whether used for academic research or real-world slope design, it helps engineers make safer, smarter decisions in challenging ground conditions.
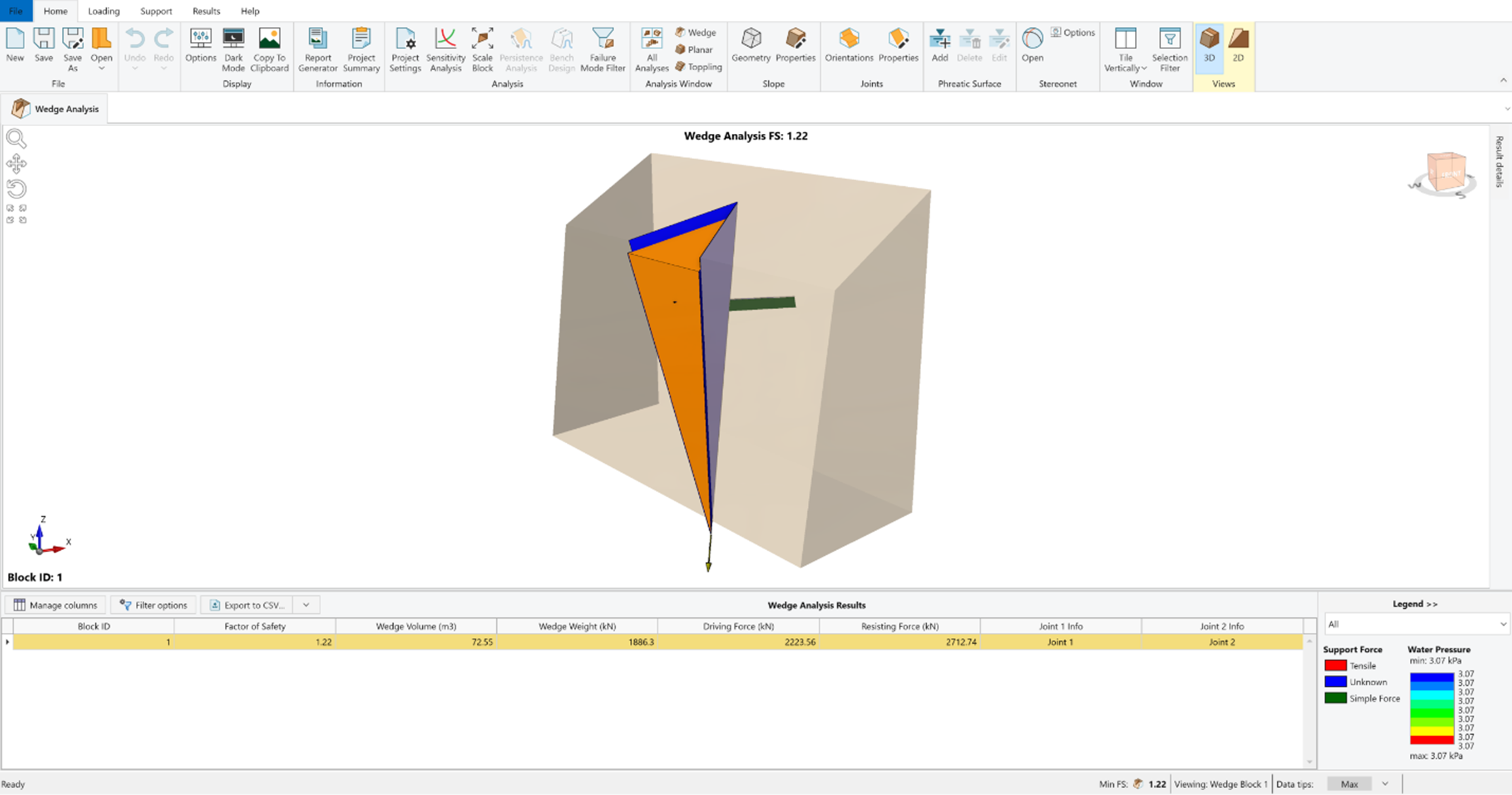